Introduction
Fusion energy, often hailed as the “holy grail” of clean energy, has seen significant advancements in recent years. Unlike conventional nuclear fission power, which splits heavy atomic nuclei to generate energy, fusion mimics the sun’s process by merging lighter elements, such as hydrogen, to create helium, thereby unleashing energy.[1][2]
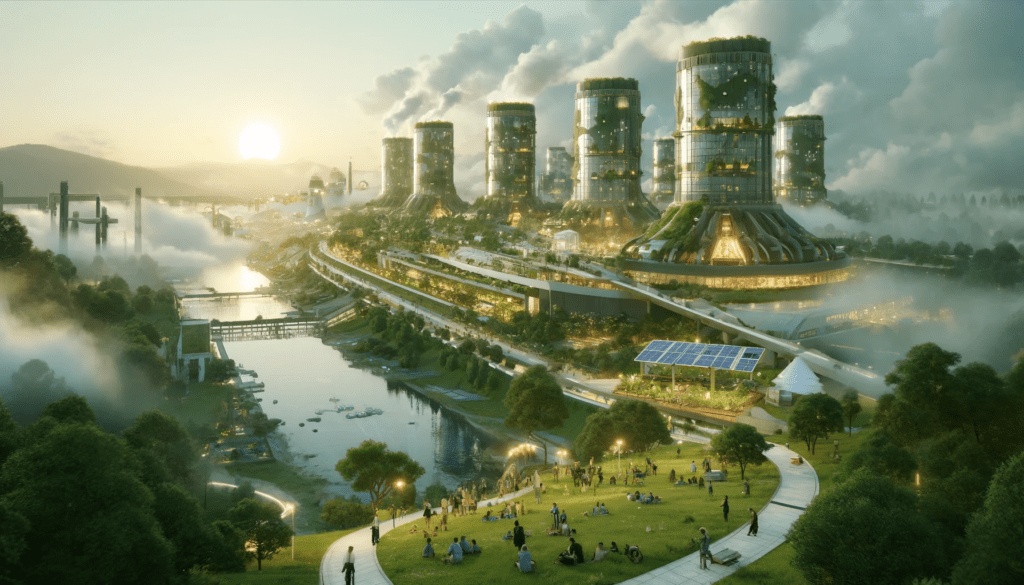
For decades, the world has relied on fission energy to meet its growing energy demands. While fission nuclear power plants have provided vast amounts of electricity, they come with significant drawbacks. The process produces highly radioactive waste. It remains hazardous for millions of years due to its extremely slow decay rate. The decay rate of radioactive materials refers to the time it takes for half of a radioactive substance to lose its radioactivity, known as the half-life. Fission by-products, such as plutonium-239, have half-lives of 24,100 years, others even millions of years, posing risks for countless generations.[3]
Additionally, the inherent risks of fission technology have resulted in catastrophic failures. The meltdowns at Chernobyl (1986) and Fukushima (2011) exposed the severe flaws in relying on fission energy.[4][5] The fallout from these disasters killed people, devastated ecosystems, displaced communities, and led to long-term health consequences. In 2012, scientists at the Max Planck Institute for Chemistry in Mainz, Germany, have calculated that such events may occur once every 10 to 20 years — some 200 times more often than estimated in the past![6]
In contrast, fusion energy produces radioactive waste with significantly shorter half-lives. For example, tritium, a by-product of fusion reactions, has a half-life of only 12.3 years.[7] This stark difference means that fusion waste requires much less long-term management (and associated costs) and poses a drastically reduced risk to future generations.
Thus, the time has come to shift focus and increase investment into fusion technology. It generates minimal radioactive by-products, poses no risk of catastrophic meltdowns, and offers an abundant fuel supply derived from seawater and other accessible sources.
Fusion Technology
A fusion reactor works by mimicking the process that powers the sun, where lighter atomic nuclei, such as hydrogen, merge to form a heavier nucleus, like helium. This process requires extremely high temperatures, around 100 million degrees Celsius, to overcome the repulsive forces between the positively charged nuclei. At these temperatures, the fuel becomes a hot, electrically charged gas called plasma.[8] You may know plasma from neon lights or fluorescent tubes, but the plasma in these devices is much colder and is meant to create light, not heat.
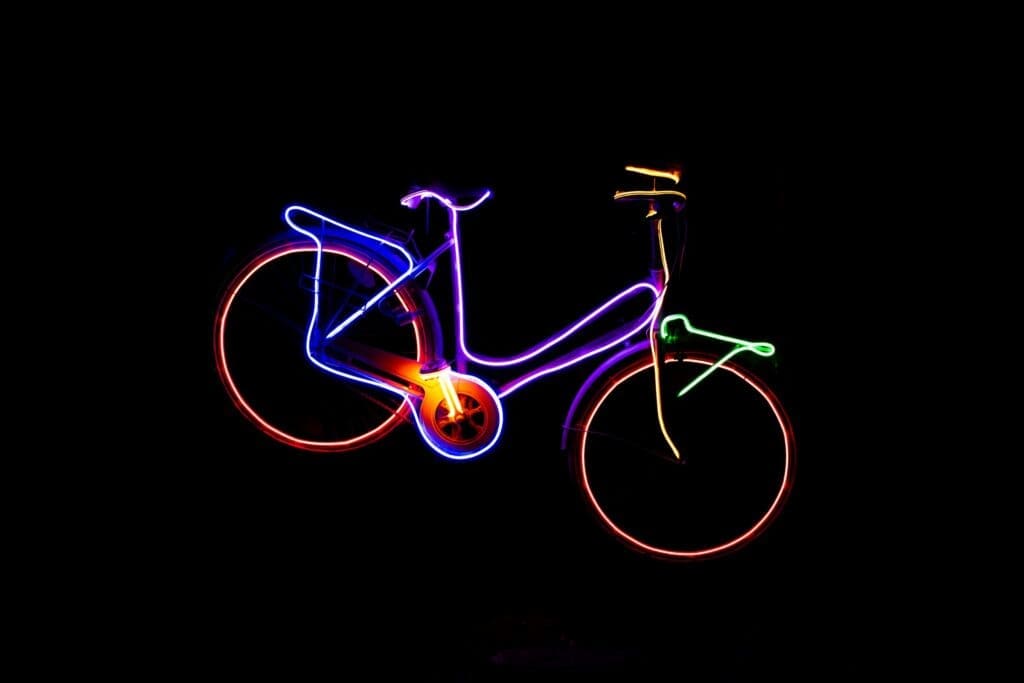
In the plasma state, the nuclei can collide and fuse, releasing a tremendous amount of energy. This energy is primarily in the form of heat, which can then be used to generate electricity through conventional power generation methods, such as driving turbines. Fusion reactors promise a nearly limitless and clean source of energy if the technical challenges can be overcome.
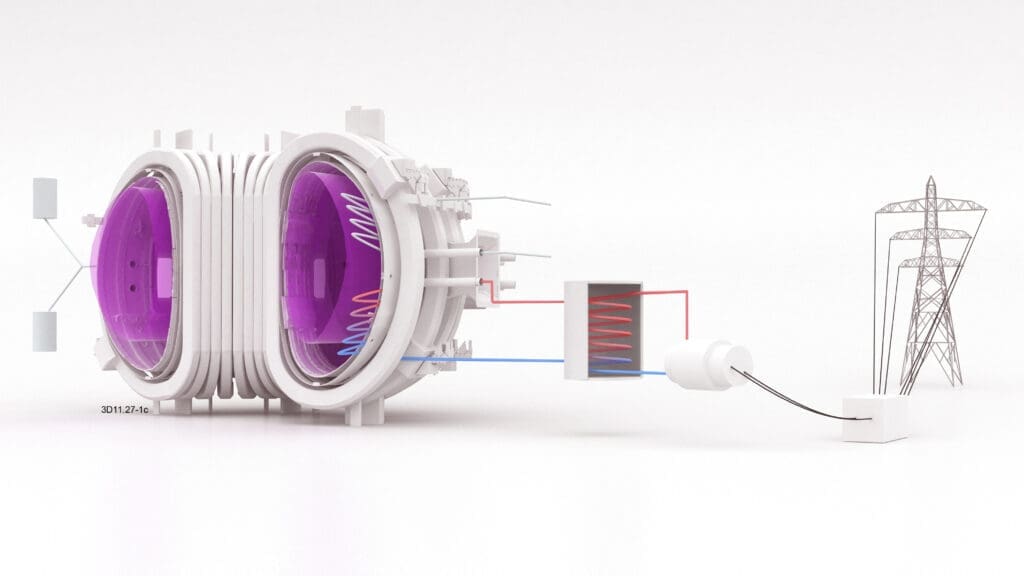
By investing in this cutting-edge technology, humanity can pave the way for a cleaner, safer, and more sustainable energy future for all inhabitants on the planet. By embracing these Solarpunk principles, fusion energy projects can contribute to efficiently minimize environmental impact.
History of Fusion Energy
The journey of fusion energy began in the early 20th century, with British physicist Francis William Aston’s discovery in 1920 that the mass of four hydrogen atoms is greater than that of one helium atom.[1] This implied that energy could be released by combining hydrogen atoms to form helium. The 1950s saw the development of the tokamak and stellarator concepts, which became the foundation for modern fusion reactors.[1]
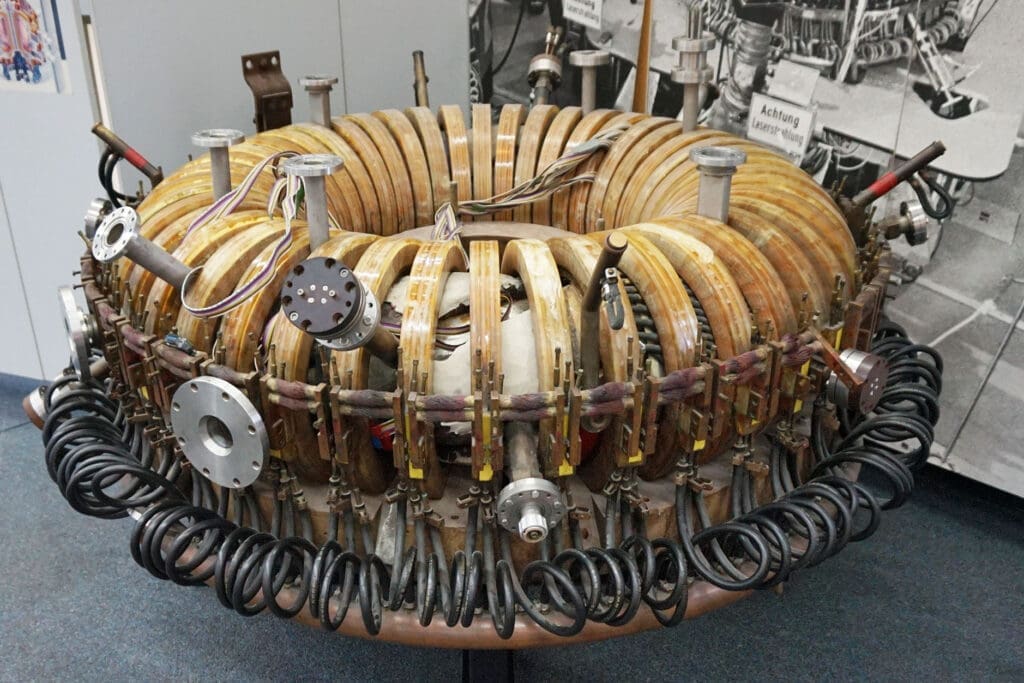
“Doughnut” shaped tokamaks and stellarators are devices designed to achieve nuclear fusion by confining extremely hot plasma with magnetic fields. Magnetic fields are essential to keep the plasma, a superheated gas where atomic nuclei can collide and fuse, from touching the reactor walls and cooling down. Tokamaks use a combination of circular magnetic fields and electric currents within the plasma to create a twisting field that holds the plasma in place, though this reliance on currents can lead to instability.[9]
Stellarators avoid this issue by using carefully shaped external magnets to create the twisting fields, making them more stable and capable of continuous operation, though they are harder to build. Advances in computational modeling and engineering are giving the stellarator reactors new boost and narrow the gap between the two concepts.[10]
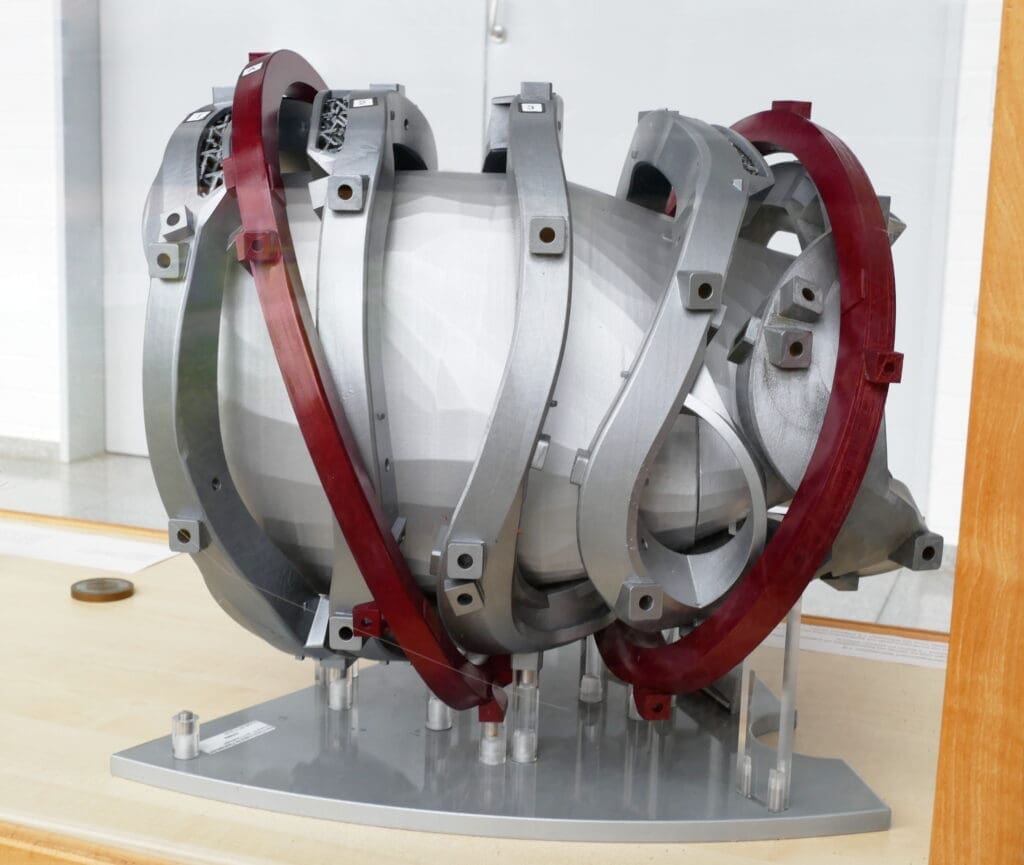
Top Teams and Countries Working on Fusion Energy
ITER (International Thermonuclear Experimental Reactor), France: A collaborative project involving the European Union, the United States, Russia, China, India, Japan, and South Korea. ITER aims to demonstrate the feasibility of fusion as a large-scale and carbon-free source of energy.[11]
ITER is a groundbreaking fusion experiment that relies on tokamak based magnetic confinement, where superconducting magnets control and confine plasma in a doughnut-shaped chamber. ITER will use a mixture of deuterium and tritium, two isotopes of hydrogen, as its fusion fuel. When these nuclei fuse, they produce helium and a neutron, releasing a large amount of energy in the process.
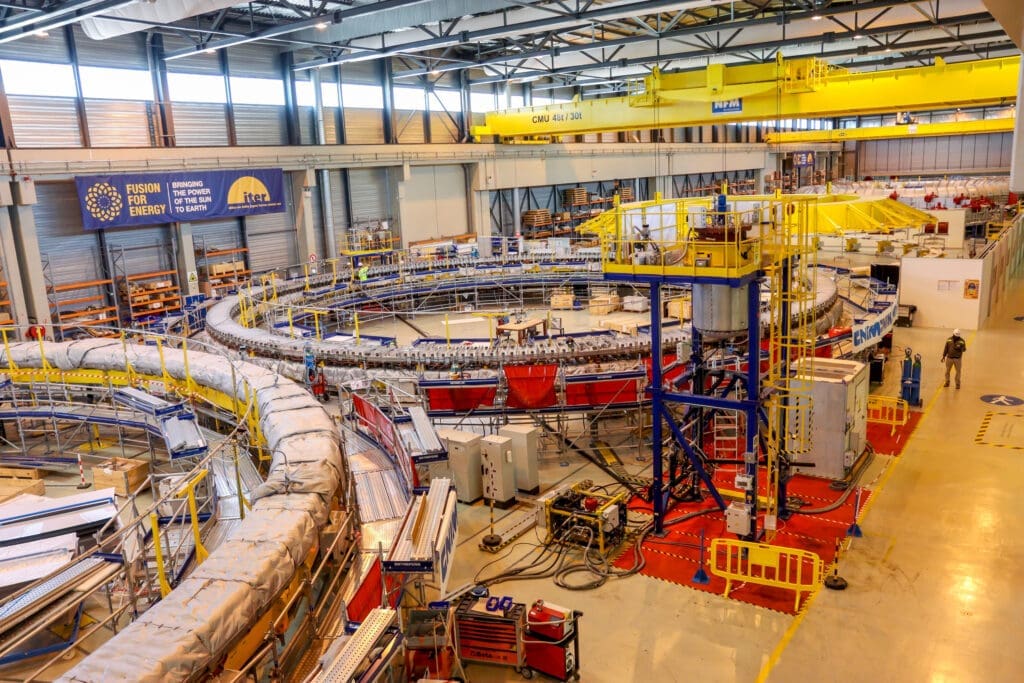
The purpose of ITER is to demonstrate the feasibility of large-scale fusion power. It aims to produce 500 MW of output power with only 50 MW of input, achieving a power gain (Q) of 10, a major milestone in fusion energy research. The project also seeks to test critical technologies needed for future reactors, such as continuous plasma operation and systems for breeding tritium fuel. By achieving these goals, ITER will pave the way for the development of commercial fusion power plants.
The decision to use a tokamak design for ITER is based on its long track record and proven efficiency in confining plasma. While tokamaks face challenges such as instabilities and reliance on pulsed operation, they remain the most advanced and viable option for scalable fusion energy production.
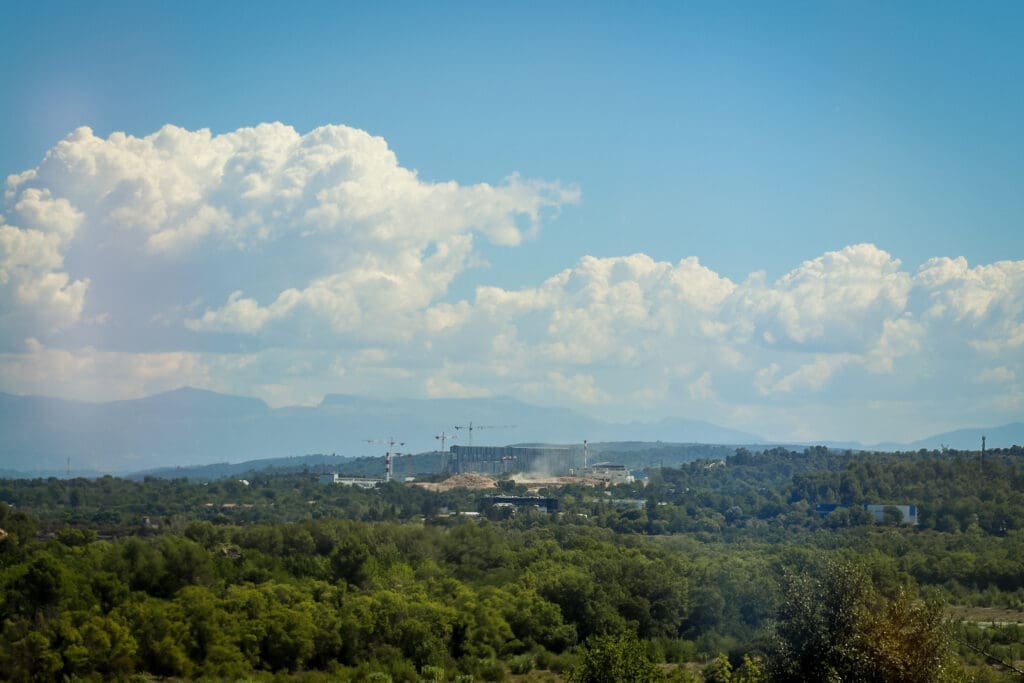
Commonwealth Fusion Systems (USA): This startup, spun out of the MIT in 2018, is focused on developing compact fusion reactors using high-temperature superconductors (HTS). Their project, SPARC, aims to achieve net energy gain from fusion, utilizing HTS magnets for a smaller, more efficient reactor design. Following SPARC’s success, CFS plans to build ARC, a commercial fusion power plant capable of generating hundreds of megawatts of clean electricity.
CFS is also advertising to have the first commercial fusion reactors available in the early 2030s. They are planning to build the world’s first grid-scale fusion power plant in Chesterfield County, Virginia.[9] The plant, based on a tokamak fusion reactor design and named ARC (= Affordable, Robust, Compact), is expected to generate about 400 megawatts of clean, carbon-free electricity, enough to power large industrial sites or about 150,000 homes.[12]
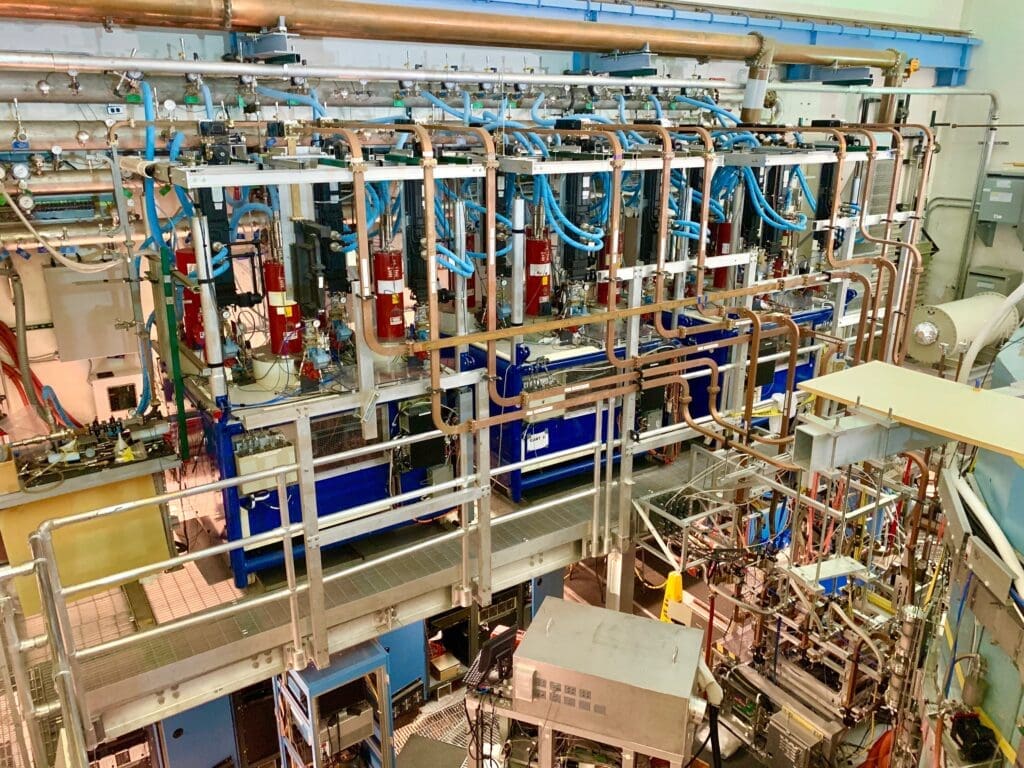
General Fusion (Canada) is pioneering a magnetized target fusion approach to bring fusion energy to the market by the end of the decade. Their innovative technique involves using powerful pistons to compress plasma and achieve the conditions necessary for fusion. This method promises a more practical and cost-effective path to commercial fusion energy.[13]
Costs and Efficiency
The cost of fusion energy is currently high due to the complexity and scale of the projects. ITER, for example, has a budget of over €20 billion (approximately $22 billion). However, as technology advances and more reactors are built, costs are expected to decrease. The efficiency of fusion reactors is measured by their ability to produce more energy than they consume. Current experimental reactors have achieved net energy gain, but commercial reactors are still a few decades away.
In February 2024, the Joint European Torus (JET) facility in the UK achieved a groundbreaking milestone by setting a new world record for fusion energy output. During its final deuterium-tritium experiments, JET produced 69 megajoules of energy from just 0.2 milligrams of fuel over a five-second period.[14] This remarkable achievement demonstrated the potential of fusion energy for future power plants. The success of these experiments provides valuable insights and boosts confidence in the development of commercial fusion reactors.
Outlook for the Next 50 Years
Over the next 50 years, fusion energy is expected to evolve significantly. Advances in materials science, superconductors, and plasma physics will drive improvements in reactor design and efficiency. Cold fusion, a controversial and less understood form of fusion, may also see breakthroughs if researchers can overcome current skepticism and technical hurdles. The goal is to achieve commercial fusion reactors that provide a reliable and sustainable source of clean energy, contributing to global decarbonization efforts.
Conclusion
Fusion energy holds great promise for a sustainable future, aligning with solarpunk ideals of eco-friendly, clean energy. While challenges remain, ongoing research and international collaboration show significant progress, paving the way for a future powered by fusion energy.
Sources:
[1] https://en.wikipedia.org/wiki/History_of_nuclear_fusion
[2] https://www.britannica.com/science/nuclear-fusion/History-of-fusion-energy-research[3]
Plutonium-239 – Wikipedia
[4] https://www.iaea.org/topics/chornobyl
[5] https://www.unscear.org/unscear/en/areas-of-work/fukushima.html
[6] https://phys.org/news/2012-05-probability-contamination-severe-nuclear-reactor.html
[7] Tritium – Wikipedia
[8] https://en.wikipedia.org/wiki/Fusion_power
[9] https://en.wikipedia.org/wiki/Tokamak
[10] https://en.wikipedia.org/wiki/Stellarator
[11] https://www.iter.org
[12] https://cfs.energy/news-and-media/commonwealth-fusion-systems-to-build-worlds-first-commercial-fusion-power-plant-in-virginia
[13] https://generalfusion.com/post/category/press-releases/
[14] JET’s final tritium experiments yield new fusion energy record – GOV.UK